Some companies and organisations research and use gene drive technology in mosquitoes as a tool against malaria
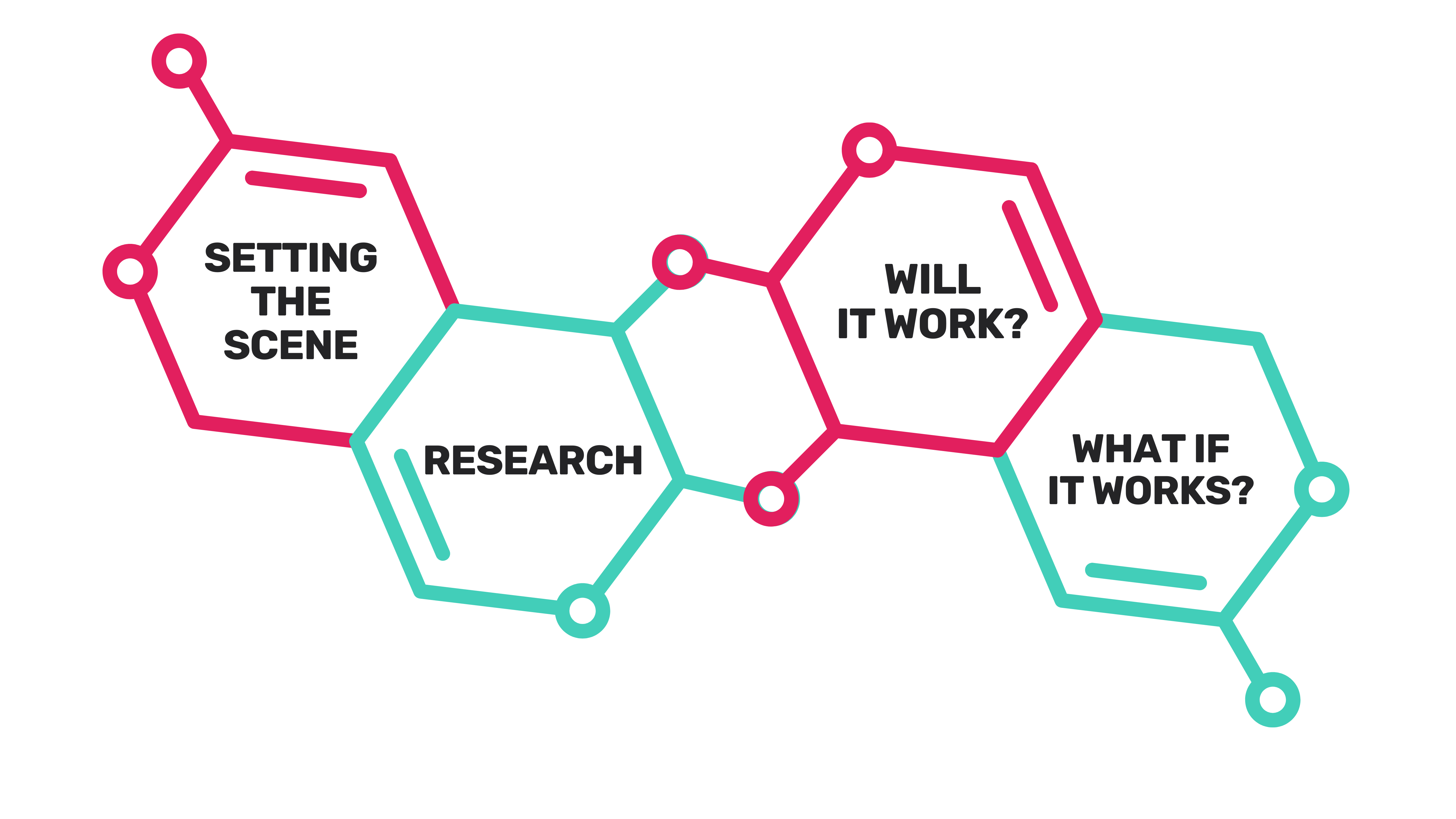
Setting the scene
Gene drives and malaria
Malaria is one of the leading causes of disability and death in Africa. 2020 saw 241 million cases and 627,000 deaths worldwide. 95% of these deaths were in Africa, with 80% of them occurring in those under five year olds. Together with the devastating health effects, it also accounts for half of missed school days in Africa. Malaria is caused by the microscopic parasites of the Plasmodium species, which infect the red blood cells. It is transmitted by female Anopheles mosquitoes, and is passed from person to person via the mosquito feeding on the blood of an infected person and then passing on the Plasmodium parasite. The first symptoms are fever, headaches and chills occurring around 10-15 days after infection, and it can progress to severe illness and death within 24 hours of symptoms or can run a more chronic course¹.
Gene drive technology is being actively researched and is planned to be used in mosquitoes as a tool against malaria. The furthest developed gene drive in mosquitoes is in the species of Anopheles gambiae s.s, the main vector of malaria in sub-Saharan Africa. Gene drive technology has been proposed for malaria in a variety of forms, but the one that has been most invested in and is furthest developed is the suppression drive. A suppression drive means the use of the gene drive with the aim of eliminating the malaria-carrying mosquito, in this case by passing down a gene with the aim to prevent each new generation of females from being born, or causing them to develop as intersex and infertile and thus leading to a die off of the population. The use of the gene drive ensures that the genetic trait responsible for this mutation can be passed down to each new generation at an unusually high rate of inheritance (close to 100%), meaning each new generation should carry it. Though the exact numbers are disputed, models suggest that the use of gene drives could decrease the Anopheles gambiae numbers significantly if the gene drive functions as planned2.
Target Malaria
One of the main organisations developing gene drives against the malarial mosquito is Target Malaria, a not-for-profit organisation which has been involved in developing genetic methods for vector control since 2005. Target malaria is active in 4 countries in sub-Saharan Africa including Burkina-Faso, Mali, Ghana and Uganda and has research laboratories in the UK, Italy and the USA. Most of the research happens at their partner laboratory at Imperial College London. Target Malaria is working on gene drive constructs in the three major vectors of Malaria in sub-Saharan Africa; A. gambiae s.s, A. funestus and A. coluzzi. While the originally intended release point of 2024 has been pushed back, the stated aim of Target Malaria is to release gene drive mosquitoes in Africa within the next 10 years.
The organisation focuses on three areas with different teams: ‘research’, ‘regulatory science’ and ‘stakeholder engagement’. Simply put this means their aims are to study and develop gene drives, research how to design and carry out risk assessments, and to engage with local groups of people in the areas where release is being considered. The rationale behind Target Malarias work is, according to them, that previous attempts at reducing the disease burden have been unsuccessful and that gene drives, in combination with other existing methods of control, could lead to the eradication of malaria.
They acknowledge to some extent certain unknowns of gene drive technology, but insist that the problem of malaria is so urgent and unsolvable with the means that we currently have, that we must use gene drive technology. Problems associated with the new technology may arise in the course of this use, but the issue is so pressing that we must learn as learn as we go.
1 Centers for Disease Control and Prevention. (2022, March 22). CDC – Malaria – about malaria – faqs. Centers for Disease Control and Prevention. Retrieved October 29, 2022, from https://www.cdc.gov/malaria/about/faqs.html
2 North, A.R., Burt, A. & Godfray, H.C.J. Modelling the suppression of a malaria vector using a CRISPR-Cas9 gene drive to reduce female fertility. BMC Biol 18, 98 (2020). https://doi.org/10.1186/s12915-020-00834-z
Shedding light on Target Malaria
Interview with Ali Tapsoba and Guy Yaméogo
Burkinabe civil society activists Ali de Goamma Tapsoba and Guy Yameogo discuss their concerns about the lack of transparency surrounding Target Malaria’s experimentation in Burkinabe villages, inadequate stakeholder engagement with the villagers, the lack of free, prior and informed consent, and unethical practices of Target Malaria.
Research
Types of gene drive in mosquitoes
In the past, two types of gene drives have been proposed as the best candidates in the fight against malaria. The first involves a ‘modification drive’– this is where a ‘cargo’ or ‘payload’ gene is inserted into the gene drive construct in the mosquito which it passes on at a rate of close to 100% every time it breeds, with the aim of totally replacing the wild population with mosquitoes that have the desired characteristic- for example, a gene that codes for resistance against Plasmodium parasites, preventing the parasite from developing in the mosquito and therefore preventing it from spreading the infection. The aim would therefore be to have a modified, malaria parasite-resistant population of Anopheles that cannot pass on the disease. The other proposition is a ‘suppression drive’, where the gene drive is designed to eradicate or drastically reduce numbers of Anopheles mosquitoes through removing genes essential to their survival or reproduction, leading to their death or abnormal development. The furthest developed example of this involves killing off the females from the population so that the population crashes. The aim of this would be to reduce the malaria burden through eliminating or vastly reducing the number of Anopheles mosquitoes from the area, so there is no vector to pass on the disease1.
Target malaria’s research
Target Malaria have opted to develop and implement the suppression drive and aim to eradicate or drastically reduce the Anopheles gambiae s.s mosquito. Together with its partner laboratory at Imperial College London, the organisation is developing and trialling such suppression drives. One of the constructs developed targets the so-called doublesex gene, an integral gene to development of sexual organs- male mosquitoes inheriting this drive should develop normally, while the females should develop as intersex, which cannot bite or lay eggs. The wild-type doublesex gene is so important that any change to it is likely to affect the mosquito’s survival and will be selected against- in evolutionary biology, this type of gene is called ‘highly conserved’. This means that the mosquitoes are less likely to develop a so-called ‘resistance’ (see: ‘will it work’ section) to the gene drive and therefore it is more likely to be able to spread unfettered throughout the intended populations with nothing inhibiting its spread. Another gene drive construct is aimed at two targets- it affects both the doublesex gene and destroys or ‘shreds’ the X chromosome (a so-called ‘X-shredder construct’) in the sperm that carry it, leading to a male only population as females are prevented from being born. The reason to use two mechanisms is that if one mechanism fails, the other one theoretically should take over2.
As far as is currently known, the combined-target gene drive mosquito with both the X-shredder and doublesex gene disruptor is the one intended for use by Target Malaria. This construct has been engineered in the Anopheles gambiae s.s mosquito, the primary vector of malaria in Africa (as previously described in our work however, this gene drive is highly likely to spread throughout the highly similar Anopheles gambiae complex of 9 mosquito species). Both gene drive constructs have been shown to work at the early stages in laboratory cages, completely crashing populations of A. gambiae s.s within less than a year3,4. The doublesex construct was later tested in larger cages in Italy that allow for more realistic mating conditions5, and similar trials are planned for the combined doublesex and X-shredder construct.
Plans for release
Target Malaria had originally aimed to release gene drive mosquitoes carrying the doublesex and x-shredder, most likely at their site in Burkina-Faso, in 2024. However, the project is delayed and the first release is planned in the next 10 years. Meanwhile they are slowly working towards the release of gene drive mosquitoes by conducting trials on genetically modified non gene drive mosquitoes, building infrastructure and lobbying governments and local populations. They have recently finished renovations on the Arthropod Containment Level (ACL-2) insectary, which currently houses genetically engineered (non-gene drive) male-bias mosquitoes, which cause offspring to be born at a rate of around 95% male in the laboratory. Target Malaria are seeking approval for a trial release in nature. They have recently published the results from a study whereby they released genetically-engineered (but not gene drive) sterile male mosquitoes. These releases are done with the intention of modelling the dynamics of and collecting further data on how gene drive mosquitoes are likely to behave in wild populations before releasing gene drives mosquitoes.
Little discussed is the spectrum of risks arising if it doesn’t work as advertised- there are many potential outcomes between the gene drive succeeding in its design and eradicating the target mosquito population, and total failure whereby it has no effect. These are a different but related set of risks involving the unprecedented introduction of a novel, untested, powerful and currently irreversible technology that lingers long term in a complex and insufficiently studied ecological system.
1 ENSSER (2019) GENE DRIVES: A report on their science, applications, social aspects, ethics and regulations. Critical Scientists Switzerland (CSS).
2,3 Simoni, A., Hammond, A.M., Beaghton, A.K. et al. A male-biased sex-distorter gene drive for the human malaria vector Anopheles gambiae. Nat Biotechnol 38, 1054–1060 (2020). https://doi.org/10.1038/s41587-020-0508-1
4 Kyrou, K., Hammond, A., Galizi, R. et al. A CRISPR–Cas9 gene drive targeting doublesex causes complete population suppression in caged Anopheles gambiae mosquitoes. Nat Biotechnol 36, 1062–1066 (2018). https://doi.org/10.1038/nbt.4245
5 Hammond, A., Pollegioni, P., Persampieri, T. et al. Gene-drive suppression of mosquito populations in large cages as a bridge between lab and field. Nat Commun 12, 4589 (2021). https://doi.org/10.1038/s41467-021-24790-6
Will it work?
Intro
Much attention is (rightfully) given to the ecological risks if gene drive mosquitoes succeed in their eradication mission. However, little discussed is the spectrum of risks arising if it doesn’t work as advertised- there are many potential outcomes between the gene drive succeeding in its design and eradicating the target mosquito population, and total failure whereby it has no effect. These are a different but related set of risks involving the unprecedented introduction of a novel, untested, powerful and currently irreversible technology that lingers long term in a and insufficiently studied ecological system, together with a first of its kind use of CRISPR technology embedded in the genome of an animal with the intention to spread into the wild. Thus, exploring the question of whether gene drives in mosquitoes are likely to succeed in their stated application or not is a fundamental question behind the risk-benefit calculation and should be a key part of the risk assessment and regulatory process.
In this section we will explore the mechanisms behind and potential outcomes resulting from the gene drive mosquitoes not working as planned, touching on the particular risks associated with this.
Resistance
Will the gene drive spread successfully through the population? One possibility that may prevent this is the rise of resistance to the gene drive. Resistance can occur when the target gene in the wild organism is too different to the guide RNA/template of the gene drive, meaning the construct can’t be passed on. It can arise through multiple routes, but the most common are the following: natural variation in the target gene, new natural mutations arising from natural selection on the wild population after the gene drive is introduced or new mutations from the result of the wrong action or repair of the CRISPR-Cas9 gene drive mechanism itself. This final source of variation occurs when CRISPR cuts the chromosome at the correct site, but instead of using the gene drive construct as a template to replace the cut section with, the chromosome is simply glued together in a process called non-homologous end joining (NHEJ). If this occurs and is passed down, the gene drive is prevented from passing on to the next generation and therefore cannot spread through the population; the organism is ‘resistant’ to it1. Evolutionary biology predicts the following: individuals that are ‘resistant’ (i.e. their target gene is different enough from the one the gene drive was designed for that the gene drive cannot copy itself) will be very strongly selected for and will be favoured by evolution in comparison to the gene drive-carrying mosquitoes. This could cause the drive to not function at all after a few generations, even if there is only a small initial ‘seed’ of resistant mosquitoes2.
In the A. gambiae s.s gene drive mosquitoes which are likely to be chosen for the release in Africa, gene targets have been chosen that are less likely to mutate or be different between individuals or populations because they are so important to survival3,4. However, multiple different trials at the Target Malaria lab showed that resistance still developed despite the use of highly conserved targets5,6. Accordingly, resistance to one of the current proposed gene drive targets of doublesex cannot be ruled out from occurring7, with 2.9% of Anopheles in African countries found to have a mutation in the doublesex gene8. The researchers at Target Malaria acknowledge that resistance may arise to the gene drive once the modified mosquito is environmentally released and prevent it from working as intended9.
Different behaviour
The behaviour of gene drive mosquitoes may be so different to wild mosquitoes that they do not succeed. A key requirement for the theoretical success of gene drive mosquitoes is that they behave the same as normal mosquitoes in the wild. If not, this could have two effects: it may be a basis by which resistance to the gene drive can occur as there would be a strong selective pressure on wild mosquitoes to avoid the strangely behaving gene drive mosquitoes. Furthermore, mating behaviours between may be so different that it prevents them breeding. Behavioural differences between wild and lab-raised mosquitoes have been seen in field trials with the sterile insect technique, in which sterile male insects that have been treated with radiation are released in order to mate with wild-type females as a way to crash the population. Upon release, differences in environments between the lab and wild environments affected the behaviour and mating preferences of transgenic insects to such an extent that some insect control programs have failed due to it10,11. Target Malaria’s releases of genetically modified, non-gene drive male bias mosquitos in Burkina Faso seem to confirm this, with the results showing that the ability of the mosquitoes to survive and reproduce are between 20-30% lower than the wild type12. It is yet to be seen if this will be the case for gene drive mosquitoes in the wild, though based on prior experience with modified mosquitoes, there is no logical reason to suspect otherwise.
Furthermore, the models predicting the spread and function of gene drives are likely inaccurate representations of how mosquitoes would spread in real life. They don’t take into account complex ecological interactions such as and mating patterns.
A density-dependent effect would be where mosquito numbers are not dictated by the number of them born, but rather by other factors such as food availability, mating opportunities or competition between individuals. In simple terms, it means that decreasing the number of mosquitoes that hatch may not actually be the deciding factor in how many survive until adulthood, as they are limited by other factors. This is well established in Aedes mosquitoes13 Furthermore, most models assume totally random mating (known as panmixia). However, this is unrealistic for nature and far more commonly species mate ‘assortatively’- rather than randomly, mating occurs in clusters under the influence of complex ecological and geographical factors14. Assortative mating through different swarming patterns and times has also been observed in A. gambiae s.s species15. This difference between models and reality could have huge implications for how the gene drive actually spreads and also suggests that the ecological risks cannot be assumed without much more study into these patterns of interaction.
Will they work well enough to survive and breed?
Another factor which may stop the gene drive mosquitoes integrating into the population is that heterozygous carriers of the gene drive (those that have one copy of the gene) in the tested mosquitoes, are significantly less biologically fit than their wild counterparts. That means that they have decreased rates of survival and egg production than the non-GD mosquitoes. This finding is thought to be due the actual presence of CRISPR or the gene drive itself. This was shown in a study by Target malaria in which the gene drive failed after only three generations due to very strong negative effects of the gene drive construct16. The mechanism is not yet completely clear, but is likely due to the genetic promoter causing gene expression to work in a different way than would be expected, with CRISPR-Cas9 being active also in the normal body cells (called somatic cells), rather than as it is designed to in the sexual cells shortly after reproduction17. CRISPR working in body cells could also increase the number of mutations that are caused by the gene drive itself, as the wrong repair mechanism is more often used in these cells- instead of copying from the gene drive template through a mechanism called homology directed repair (HDR), a requirement for the successful use and passing on of gene drives, the two ends of the broken DNA strands are simply stuck together in a process called non-homologous end joining (NHEJ)18. It is mentioned in one study from Target Malaria that rates of HDR may be 100x higher in the sex cells compared with in somatic/body cells, meaning that the inexact repair mechanism of NHEJ dominates in the somatic cells18. As well as having the concerning effect of creating many unpredictable mutations in the mosquito, the fact of CRISPR also being active in the body cells increases the likelihood of resistance arising and of gene drive failure through NHEJ creating mutations in the target gene site and stopping the gene drive from passing itself on. The current level of unreliability of the proposed gene drive mechanisms should raise serious questions as to whether they are likely to achieve their stated aims.
Will it eradicate malaria?
According to a model by North, Burt and Godfray19 (2020), introduction of the proposed doublesex/X-shredder Gene Drive mosquito would lead to a substantial decrease of the Anopheles gambiae s.s population within 10 years. The likelihood of this is questionable – the model it is based on seems to assume random mating patterns, which we have demonstrated to not be the reality. It also relies on the assumption that any negative effect of the gene drive itself on the survival and reproduction (called ‘biological fitness’, how well the organism can pass on its genes in any given environment, or in other words how suited it is to its environment) of the modified mosquito would be limited to a maximum of 40% less than the wild type- in other words, according to the model, if the modified mosquitoes are over a threshold of 40% worse at reproducing and/or surviving than the wild type, the gene drive cannot spread. The reality of the gene drive mosquitoes in the lab shows that a fitness decrease of over 40% is very likely, with decreased fitness levels of around 80% less than the wild type mosquitoes often seen in laboratory studies of Target Malaria (in one experiment even to the extent that the gene drive failed after three generations20,21 Target Malaria’s recent releases of genetically modified, non-gene drive male bias mosquitos in Burkina Faso show that fitness costs could also be expected in the wild, with the fitness of the modified mosquitoes being between 20-30% lower than the wild type, though this is not enough to inhibit the spread according to the model mentioned above. Another factor here that may lead to failure is resistance to the gene drive- the more poorly the drive performs, the longer it is present in the population and the higher the chance of resistance evolving to the gene drive during this timescale.
Furthermore, even if the numbers of mosquitoes are significantly decreased, the question of whether reducing them will decrease the number of cases to the extent that malaria is eradicated is far from settled. Disease transmission dynamics are not always just directly related to the number of disease vectors there are. This can be seen with the phenomenon of the 80/20 rule, seen in almost every disease. To understand the 80/20 rule we can take the example of a respiratory virus, where often 20% of cases are responsible for passing on the disease to the remaining 80% of cases. A similar dynamic is also seen in the transmission of malaria22. Therefore even if we assume the modelling is correct and there will be a substantial decrease of A. gambiae mosquitoes, it is far from given that this will lead to a halt of the spread of malaria23 (naturally, it must be pointed out that this final point is likely to be a common challenge across malaria eradication, not just limited to gene drives).
Risks if it doesn’t work
In this section we have explored some of the many different ways that gene drive Anopheles mosquitoes might fail- for example through resistance, behavioural differences between wild and lab mosquitoes or decreased biological fitness of gene drive mosquitoes (or a mixture of all). But does it mean that if the gene drive fails in eradicating the Anopheles mosquito, there are no risks it runs to the environment or health? This is far from true- we are instead confronted simply with a different set of risks.
The first comes from the side effects on the effort against malaria if the gene drives fail. Perhaps the biggest risk from gene drive failure could be a lack of funding and support for the measures that will and do work against malaria, disregarded in favour of gene drives. This is a known problem in malaria eradication efforts, whereby the phenomenon of ‘donor fatigue’ has led to less support for the fight against malaria due to the long-term, expensive nature and slow visibility of results of campaigns24,25.
Another risk comes from the low level presence of gene drives in the environment over a long timescale. This is complicated to explain, but relatively simple one understood. Due to the design of gene drives, the low level and long-term persistence of gene drive mosquitoes in the environment is expected if the gene drive does not successfully replicate very well (probably due to the mechanisms explored above). In these cases, lower levels of mosquitoes carrying one copy of the gene drive could be circulating for years, while the normal wild population is somewhat taken over from it but not successfully enough to be fully replaced. The long term presence of a gene drive in the ecosystem means that there is a much higher chance over time of an unexpected consequence on a molecular or ecological level- for example, an increased probability of horizontal gene transfer (HGT) or another mechanism causing spread of the drive construct to other insects (see: ‘what are the risks if it works’ section). Though statistically unlikely on a short timescale, the longer gene drives are in the environment, the higher the chances of an originally unlikely event occurring that could have negative consequences. In the case of HGT transplanting the gene drive construct to other insect species to the extent that it could spread, current models of gene drive invasiveness indicate that such an event would only have to happen a couple of times in order for it to potentially irreversibly modify a population, with extremely low numbers able to start the genetic chain reaction if the gene drive is powerful enough26.
Furthermore, if the gene drive failed it would reveal something very concerning about the scientific basis that gene drives were approved on. It would mean that the gene drive system was not well understood by the researchers in the first place, who claimed that we have a solid understanding of the science behind it. The fact of it not succeeding as envisioned would bring up the following uncomfortable questions: what else was not predicted about how the gene drives would work? What other risks does this imply?
These examples make it clear that the risk-benefit analysis of gene drives must not only analyse the ecological risks if it wipes out Anopheles gambiae. It must also simultaneously contend with the possibilities if it totally or partially fails. The discussion must be broadened to take account of not just successful eradication of the mosquito, but the whole spectrum of other possibilities and the risks they imply together with what the failure of gene drives indicates about the scientific basis gene drives were approved on.
1,25 ENSSER (2019) GENE DRIVES: A report on their science, applications, social aspects, ethics and regulations. Critical Scientists Switzerland (CSS).
2 Price, Tom & Windbichler, Nikolai & Unckless, Robert & Sutter, Andreas & Runge, Jan-Niklas & Ross, Perran & Pomiankowski, Andrew & Nuckolls, Nicole & Montchamp-Moreau, Catherine & Mideo, Nicole & Martin, Oliver & Manser, Andri & Legros, Mathieu & Larracuente, Amanda & Holman, Luke & Godwin, John & Gemmell, Neil & Courret, Cécile & Basalova Buchman, Anna & Lindholm, Anna. (2020). Resistance to Natural and Synthetic Gene Drive Systems. Journal of evolutionary biology. 33. 10.1111/jeb.13693.
3,7,9,21 Kyrou, K., Hammond, A., Galizi, R. et al. A CRISPR–Cas9 gene drive targeting doublesex causes complete population suppression in caged Anopheles gambiae mosquitoes. Nat Biotechnol, 1062–1066 (2018). https://doi.org/10.1038/nbt.4245
4 Simoni, A., Hammond, A.M., Beaghton, A.K. et al. A male-biased sex-distorter gene drive for the human malaria vector Anopheles gambiae. Nat Biotechnol, 1054–1060 (2020). https://doi.org/10.1038/s41587-020-0508-1
5,16,20 Fuchs S, Garrood WT, Beber A, Hammond A, Galizi R, et al. (2021) Resistance to a CRISPR-based gene drive at an evolutionarily conserved site is revealed by mimicking genotype fixation. PLOS Genetics 17(10): e1009740. https://doi.org/10.1371/journal.pgen.1009740
6,18 Hammond AM, Kyrou K, Bruttini M, North A, Galizi R, et al. (2017) The creation and selection of mutations resistant to a gene drive over multiple generations in the malaria mosquito. PLOS Genetics 13(10): e1007039. https://doi.org/10.1371/journal.pgen.1007039
8 The Anopheles gambiae 1000 Genomes Consortium. Genetic diversity of the African malaria vector Anopheles gambiae. Nature, 96–100 (2017)
10,14 The effect of mating complexity on gene drive dynamics Prateek Verma, R. Guy Reeves, Samson Simon, Mathias Otto, Chaitanya S. Gokhale (2022), The American Naturalist. https://doi.org/10.1086/722157doi:
11 McInnis, D. O., D. R. Lance, and C. G. Jackson. 1996. Behavioral resistance to the sterile insect technique by mediterranean fruit fly (diptera: tephritidae) in hawaii. Annals of the Entomological Society of America 89:739–744.
12 Yao, F.A., Millogo, AA., Epopa, P.S. et al. Mark-release-recapture experiment in Burkina Faso demonstrates reduced fitness and dispersal of genetically-modified sterile malaria mosquitoes. Nat Commun, 796 (2022). https://doi.org/10.1038/s41467-022-28419-0
13 O’Neal PA, Juliano SA. Seasonal variation in competition and coexistence of Aedes mosquitoes: stabilizing effects of egg mortality or equalizing effects of resources? J Anim Ecol. 2013 Jan;82(1):256-65. doi: 10.1111/j.1365-2656.2012.02017.x. Epub 2012 Jul 24. PMID: 22823120; PMCID: PMC3480969.
15 Dabiré K.R., Sawadogo S.P., Diabaté A., Toé K.H., Kengne P., Ouari A., Costantini C., Gouagna L.C., Simard F., Baldet T., Lehmann T., Gibson G.. 2013. Medical and Veterinary Entomology, 27 (3) : p. 298-312. Assortative mating in mixed swarms of the mosquito Anopheles gambiae s.s. M and S molecular forms, in Burkina Faso, West Africa
17,18 Hammond A, Karlsson X, Morianou I, Kyrou K, Beaghton A, Gribble M, Kranjc N, Galizi R, Burt A, Crisanti A, Nolan T. Regulating the expression of gene drives is key to increasing their invasive potential and the mitigation of resistance. PLoS Genet. 2021 Jan
19 North, A.R., Burt, A. & Godfray, H.C.J. Modelling the suppression of a malaria vector using a CRISPR-Cas9 gene drive to reduce female fertility. BMC Biol, 98 (2020). https://doi.org/10.1186/s12915-020-00834-z
22 Cooper, L., Kang, S.Y., Bisanzio, D. et al. Pareto rules for malaria super-spreaders and super-spreading. Nat Commun, 3939 (2019). https://doi.org/10.1038/s41467-019-11861-y
23Bier, E. Gene drives gaining speed. Nat Rev Genet, 5–22 (2022). https://doi.org/10.1038/s41576-021-00386-0
24Meshnick SR. Malaria control: tortoises and hares. Am J Trop Med Hyg. 2015 May; 92(5):885-886. doi: 10.4269/ajtmh.15-0173. Epub 2015 Mar 16. PMID: 25778502; PMCID: PMC4426573.
26Charleston Noble, Ben Adlam, George M Church, Kevin M Esvelt, Martin A Nowak (2018) Current CRISPR gene drive systems are likely to be highly invasive in wild populations eLife doi.org/10.7554/eLife.33423.
What are the risks if it works?
Ecological
The wiping out of the A. gambiae mosquito is claimed to have minimal impact on the ecosystem by Target malaria. However, the wiping out of species almost invariably has demonstrated a devastating knock-on unexpected effect, as seen throughout the history of previous years of failed biocontrol attempts. A prime example of this was the near eradication of the sparrow in China, the victim of the ‘4 plagues’ propaganda campaign by the government of the time due to its eating of grain before harvest. However, the campaign reversed direction quickly when it was discovered that sparrows were also eating agricultural pests, and China started to actually import sparrows from Russia. Nevertheless the decimated sparrow population led to a huge decrease in crop yields and was likely a key cause of the famine during which between 30-45 million people starved between 1958-19621.
Insects are similarly one of the foundations of almost every ecosystem, carrying out not only essential functions in nutrient recycling and pollination, but also being a prime source of food for many species. The Anopheles mosquito is no exception, with the larvae sustaining spiders, fish, other insects and many other species, and the adults being prey for birds and bats among others2. Do we run the same risk with gene drive mosquitoes as with the sparrows?
The effect of removing Anopheles as a food source depends on the biomass of Anopheles compared to other mosquitoes in Africa. If the percentage is large, the removal is therefore much more likely to cause a severe ecological cascade. What percentage of the biomass of mosquitoes in Africa do Anopheles gambiae account for? There is an incredible lack of research on the ecological role of A. gambiae in the ecosystems in Africa intended for release, and therefore currently insufficient evidence to answer this most important of questions. The little research there is seems to be mostly from Target Malaria themselves. The few studies there are demonstrate an important ecological role of mosquitoes; one study Target Malaria published showed that around 95% of the larvae of A. gambiae are eaten before they develop3. Furthermore, one study showed that the number and diversity of birds and dragonflies were reduced following the use of a biological insecticide4. Pollination vital for the ecosystem and farming, is also at risk; as well as being prey for other insects and birds that are pollinators, Anopheles mosquitoes also need sugar to survive, in the form of nectar from plants. This behaviour may also play a direct role in pollination5.
The ecological risks that gene drive mosquitoes imply are not limited to animals and plants. The risk of ecological breakdown from the gene drive mosquito is one that is inextricably linked to the human livelihoods that depend on a functioning and healthy ecosystem. Contrary to the confident assertions, we are clearly far from the point where we can say that the eradication of A. gambiae presents no threat to the ecosystem or human health. Six of the species under threat (from gene drives) play either no or only minor roles in malaria transmission- just the three species A. gambiae sensu strictu, A. coluzzi and A. arabiensis are considered to be major vectors of malaria.
Interspecies spread
Target Malaria acknowledged in a recent paper that their gene drive would likely not stay limited to the one species of A. gambiae sensu stricto, but would be likely to spread to other Anopheles gambiae species. Anopheles gambiae sensu stricto, the chosen mosquito of Target Malaria, is just one member of at least nine mosquito species in the ‘Anopheles gambiae complex’ (known as A. gambiae sensu lato, i.e. ‘in the wider sense’), a family of mosquito species that look identical, some of which are well-known to interbreed and produce hybrids that are capable of breeding6. Six of the species under threat play either no or only minor roles in malaria transmission- just the three species A. gambiae sensu strictu, A. coluzzi and A. arabiensis are considered to be major vectors of malaria7,8.
Two factors make it likely that the gene drive will spread beyond A. gambiae s.s. The first is the interbreeding between members of the A. gambiae complex, meaning there is genetic exchange between the species. Second is the doublesex gene, one of the proposed targets of the gene drive- it is shared amongst the whole gambiae complex. With the combination of interbreeding and a shared gene drive target, the likelihood of the gene drive spreading to all nine A. gambiae species is very high and therefore the chance of ecologically damaging consequences is multiplied. The spread to other members of the Anopheles genus could also be possible through this same mechanism, particularly with climate change altering the ranges of mosquitoes that were historically more separated. The south-east Asian A. stephensi malarial mosquito has expanded its range fairly close to A. gambiae, to Djibouti, Somalia and Sudan9.
While the chances of breeding of the two species are not known, if it were to occur even on a low level, then the shared gene drive target would drastically increase the likelihood of the gene drive interspecies spread and therefore increase the risk of unintended ecological consequences.
The discovery of mosquitoes travelling high in the air on the wind, potentially over long distances, increases these chances drastically and means the isolation of gene drive mosquitoes to one area is practically non-existent10.
The phenomenon of horizontal gene transfer (HGT) poses another risk of interspecies spread, perhaps even to other insects than mosquitoes. Horizontal gene transfer is when genes are passed between species in a method similar to infection, usually facilitated by viruses and bacteria. This seems an unlikely possibility to imagine, but if it were to occur the consequences could be disastrous for the insect kingdom- the doublesex gene is found everywhere in the insect kingdom, meaning every insect is theoretically vulnerable to the gene drive should it be passed on by an event of HGT11. Far from being a rare phenomenon, HGT is ubiquitous in the animal kingdom; a recent study examined 218 genomes from different insects and identified at least 741 separate gene transfers which transferred around 1410 genes12. The main insects affected were moths, but it also affected butterflies, beetles and wasps among others- all insects that are likely to share the same gene drive target, the doublesex gene. Whole genome sequencing of humans even suggests that 145 ‘human’ genes resulted from this process of HGT, and up to half of bacterial genes in the microbiome13. A paper modelling the likelihood of HGT passing on a gene drive to the extent that it successfully works in another species, showed that this is a realistic possible outcome of a gene drive being released into the environment14. The extent of the risk is not yet known, but until more research is carried out on it, it should be a factor to consider. A factor that is strongly linked to this is that of monitoring the gene drive once it is released into nature. Even though the costs and infrastructure to do this will be demanding, it would be essential to do so as this would be key to picking up on events such as HGT and other possible consequences of the gene drive release. So far this topic has not been adequately addressed- it should be, and as a matter of priority.
No going back
There is currently no tested mechanism to reverse it, should gene drive mosquitoes be released. The proposed mechanisms to stop this include the concept of a daisy chain drive, where the drive elements are situated on different chromosomes and each requires the other to work. This concept is currently entirely theoretical. In addition, it does not prevent the gene drive from crossing borders of different countries.
The other solution is to simply release another gene drive to replace the original one. However, besides also being untested and hypothetical, this will not restore the wild type mosquito, rather it would replace it with another modified one with no way to un-modify the population. Therefore if an unforeseen consequence results simply from the engineering techniques themselves or from CRISPR/Cas9, this would just exacerbate the problem. As CRISPR/Cas9 is not naturally present in any animal and thus is entirely evolutionarily novel15. The likelihood of problems relating to the construct itself would therefore not be surprising, as the lack of CRISPR mechanisms in any animal suggests it is absent for a reason.
Without a reliable way to recall gene drives, Kevin Esvelt, who developed the idea of using CRISPR in the gene drive mechanism, described this risk with the following; “The bottom line is that making a standard, self-propagating CRISPR-based gene drive system is likely equivalent to creating a new, highly invasive species: both will likely spread to any ecosystem in which they are viable…”16.
Unexpected effects on malaria dynamics
Another issue here is the dynamics of immunity to malaria in the African population. In the case that the drive functioned to reduce by as much as 90% the population of Anopheles, developers acknowledge that small pockets would be likely to remain. Malarial immunity functions as a complex ebb and flow based on exposure to the disease and resistance, and a sudden reduction in exposure could have the unintended consequence of actually increasing susceptibility and vulnerability of the population to malaria due to the decrease in the rate of population level immunity through exposure, meaning people are more at risk of severe malaria in later years if a bounce back in malaria transmitting mosquitoes were to occur17,18.
1 Elhassan, K. (2022) Sparrow extermination, Lantern Shields, and more terrible plans in history, History Collection. Available at: https://historycollection.com/sparrow-extermination-lantern-shields-and-more-terrible-plans-in-history/ (Accessed: October 29, 2022).
2 ENSSER (2019) GENE DRIVES: A report on their science, applications, social aspects, ethics and regulations. Critical Scientists Switzerland (CSS).
3 Collins CM, Bonds JAS, Quinlan MM, Mumford JD (2019). Effects of the removal or reduction in density of the malaria mosquito, Anopheles gambiae s.l., on interacting predators and competitors in local ecosystems. Med Vet Entomol 33:1.
4 Jakob C, Poulin B (2016). Indirect effects of mosquito control using Bti on dragonflies and damselflies (Odonata) in the Camargue. Insect Conservation and Biodiversity 9:161.
5 Foster WA (1995). Mosquito sugar feeding and reproductive energetics. Annu Rev Entomol 40:443
6 John B. Connolly, Jörg Romeis, Yann Devos, Debora C.M. Glandorf, Geoff Turner, Mamadou B. Coulibaly, Gene drive in species complexes: defining target organisms, Trends in Biotechnology, 2022
7 Anopheles gambiae (African malaria mosquito, Mosquito, Malaria mosquito, ANOGA) | BCH-ORGA-SCBD-260392 | Organism | Biosafety Clearing-House (Correct as of September, 2022)
8 Sinka, M.E., Bangs, M.J., Manguin, S. et al. The dominant Anopheles vectors of human malaria in Africa, Europe and the Middle East: occurrence data, distribution maps and bionomic précis. Parasites Vectors 3, 117 (2010). https://doi.org/10.1186/1756-3305-3-117
9 Mnzava, A., Monroe, A.C. & Okumu, F. Anopheles stephensi in Africa requires a more integrated response. Malar J 21, 156 (2022). https://doi.org/10.1186/s12936-022-04197-4
10 LEHMANN , T. et al. (2018): Wind-borne migration of mosquitoes and pathogens. Potential
for bio-surveillance. ESA, ESC and ESBC joint annual meeting, Vancouver, November 14, 2018
11 Price, D., Egizi, A. & Fonseca, D. The ubiquity and ancestry of insect doublesex. Sci Rep 5, 13068 (2015). https://doi.org/10.1038/srep13068
12 Yang, Li & Liu, Zhiguo & Liu, Chao & Shi, Zheyi & Pang, Lan & Chen, Chuzhen & Chen, Yun & Pan, Ronghui & Zhou, Wenwu & Chen, Xue-Xin & Rokas, Antonis & Huang, Jianhua & Shen, Xing-Xing. (2022). HGT is widespread in insects and contributes to male courtship in lepidopterans. Cell. 10.1016/j.cell.2022.06.014.
13 Jeong, H., Arif, B., Caetano-Anollés, G. et al. Horizontal gene transfer in human-associated microorganisms inferred by phylogenetic reconstruction and reconciliation. Sci Rep 9, 5953 (2019). https://doi.org/10.1038/s41598-019-42227-5
14 Courtier‐Orgogozo, V. et al. (2020) “Evaluating the probability of crispr‐based gene drive contaminating another species,” Evolutionary Applications, 13(8), pp. 1888–1905. Available at: https://doi.org/10.1111/eva.12939.
15 Voets O, Tielen F, Elstak E, Benschop J, Grimbergen M, Stallen J, Janssen R, van Marle A, Essrich C. Highly efficient gene inactivation by adenoviral CRISPR/Cas9 in human primary cells. PLoS One. 2017 Aug 11;12(8):e0182974. doi: 10.1371/journal.pone.0182974. PMID: 28800587; PMCID: PMC5553774.
16 Esvelt KM, Gemmell NJ (2017) Conservation demands safe gene drive. PLOS Biology 15(11): e2003850. https://doi.org/10.1371/journal.pbio.2003850
17 Cai, Li-Ming & Li, Zhaoqing & Liu, Jinliang. (2019). Modeling and analyzing dynamics of malaria transmission with host immunity. International Journal of Biomathematics. 12. 10.1142/S1793524519500748.
18 ENSSER (2019) GENE DRIVES: A report on their science, applications, social aspects, ethics and regulations. Critical Scientists Switzerland (CSS).
The fight against malaria
The assertion that everything else has been tried for malaria and gene drive is the missing piece of the puzzle is an often repeated claim by gene drive proponents. Even if gene drives were a fully developed and tested solution, rather than a rudimentary prototype with many flaws, the claim that nothing else works anymore is still not credible. Consider the fact that 40 countries have been declared malaria free- most recently Sri Lanka (2016), Paraguay (2018), Algeria (2019) and El Salvador (2021). This was achieved through a collective and sustained approach with a multitude of solutions- ones that addressed the root causes causing malaria to persist. Among others, this involved increasing laboratories for diagnosis and detection, investment in healthcare workers and reporting systems, sewage and standing water management, insecticide and bed nets and treatments. Illustrating this is the fact that the malaria burden was halved in Africa between 2000-2015, attributed mostly to the use of treatments1,2. The discussion of these facts is often left out of the debate around gene drives, in favour of a more pessimistic picture of our past and present malaria eradication efforts.
The situation of malaria in Africa is complicated. We are not asserting here that the solutions are clear, but rather wish to show that there is a complex tapestry of root causes to the issue of malaria in Africa. Together with this is a broad range of tools, some new and some old, that have proven themselves effective in the long term.
In contrast, many existing approaches to malaria have the potential (after some tweaking) not just to reduce malaria, but to positively affect many facets of the environment and health of local communities without running as many risks of complications and design failure as gene drives. Here we will present some further reading into a small selection of the range of newly developed and older methods that show strong promise or have already demonstrated efficacy against malaria.
Vaccine
An extremely promising development with a serious chance of eradicating malaria is the malaria vaccine. The research on vaccine-based approaches to malaria has recently led to many breakthroughs after decades of challenges. Mosquirix was approved in October 2021 by the WHO as the first vaccine for malaria3. Though this was an exciting development, the vaccine only has a 30% level of protection against severe disease and death for 4 years. However, a recent study showed that when it is used as a seasonal, yearly vaccine in combination with chemoprevention, it lowered deaths from malaria by 70%4. The newer R21 vaccine has recently also shown an extremely promising 80% relative reduction in severe disease and death in phase 2 trials, bypassing for the first time the minimum 75% efficacy boundary imposed by the WHO5. Following ongoing phase three trials, there are hopes that it will be on the market by 2023. Professor Adrian Hill of the University of Oxford has stated that he believes the vaccine could reduce deaths by 70% by 2030 and eradicate it by 20406. Though the results are not yet in from the larger trials, the development certainly marks a huge step forwards in reducing or eliminating malaria in Africa. It raises the question of how progress could be increased when combining this new vaccine with existing treatments and other interventions. Read more about it here (https://www.stop-genedrives.eu/en/a-new-vaccine-against-malaria/).
Rather than a lack of pharmaceutical or technological interventions, a range of studies point to a key role of socioeconomics in the persistence of malaria in Africa. This research dates back for many years, and has consistently highlighted a relationship between socioeconomic status, gender roles, education, housing quality and many other factors in the susceptibility to malaria.
Artemesinin
Another existing solution worthy of much more investment and research is that of Artemesinin combination drugs. Isolated and developed by the 2012 Nobel-winning Chinese chemist Tu Youyou from Artemisia annua, a plant used for thousands of years in Chinese medicine, Artemesinin was largely responsible for the halving of malaria rates in Africa over the past 20 years7. Gomes et al. reported a 96% decrease in malaria deaths in Zambia using artemesinin suppositories and a halving of parasite load in 12 hours8. Reports of resistance to Artemesinin have been arising in recent years, particularly in eastern Asia. However, how much of a threat this is, is strongly debated in the malaria research community. Research at St. George’s University London, elucidating the mechanism of artemesinin, suggests that resistance is actually very unlikely to arise, due to the difficulty of Plasmodium parasites simultaneously being resistance to the extremely large range of receptors that artemesinin’s parasite-killing effect is mediated through9. Further research backs this up, suggesting that resistance to the drugs is actually to the drugs that artemesinin is paired with in the combination therapy, and that the efficacy of artemesinin remains extremely high when paired with the right drug10,11,12. A recent review highlights this, coming to the conclusion that artemesinin remains the best drug available against malaria13.
Socioeconomics, inequality and malaria
Rather than a lack of pharmaceutical or technological interventions, a range of studies point to a key role of socioeconomics in the persistence of malaria in Africa. This research dates back for many years, and has consistently highlighted a relationship between socioeconomic status, gender roles, education, housing quality and many other factors in the susceptibility to malaria.
Read more about it in these studies:
Tusting LS, Willey B, Lucas H, Thompson J, Kafy HT, Smith R, et al. Socioeconomic development as an intervention against malaria: a systematic review and meta-analysis. Lancet. 2013;382:963– socioeconomic development as best medicine for malaria.
This review finds that low socioeconomic status is linked to roughly a doubled risk of clinical malaria in children
Bruce-Chwatt L, de Zulueta J. The rise and fall of malaria in Europe. London: Oxford University Press, 1980.
This historical exploration illuminates the elimination of malaria from Europe, and suggests that malaria was eventually eradicated because of economic development rather than because of specific interventions
The arrival, establishment and spread of exotic diseases: patterns and predictions. Nat Rev Microbiol 2010; 8: 361–71. Custodio E, Descalzo MÁ, Villamor E, et al. Nutritional and socio-economic factors associated with Plasmodium falciparum infection in children from Equatorial Guinea: results from a nationally representative survey. Malar J 2009; 8: 225. Nutrition factor in malaria
These studies link good nutritional status to a far better protection against malaria
Lindsay SW, Jawara M, Paine K, Pinder M, Walraven GE, Emerson PM. Changes in house design
reduce exposure to malaria mosquitoes. Trop Med Int Health. 2003; 8(6):512–7. PMID: 12791056
This study demonstrates that house design (also strongly linked to socioeconomic status) has an effect on the likelihood of malaria infection
1 Shretta R, Liu J, Cotter C, et al. Malaria Elimination and Eradication. In: Holmes KK, Bertozzi S, Bloom BR, et al., editors. Major Infectious Diseases. 3rd edition. Washington (DC): The International Bank for Reconstruction and Development / The World Bank; 2017 Nov 3. Chapter 12. Available from: https://www.ncbi.nlm.nih.gov/books/NBK525190/ doi: 10.1596/978-1-4648-0524-0_ch12
2 Fang Huang, Xin-Yu Feng, Shui-Sen Zhou, Lin-Hua Tang & Zhi-Gui Xia (2022) Establishing and applying an adaptive strategy and approach to eliminating malaria: practice and lessons learnt from China from 2011 to 2020, Emerging Microbes & Infections, 11:1, 314-325, DOI: 10.1080/22221751.2022.2026740
3 World Health Organization; WHO recommends groundbreaking malaria vaccine for children at risk; News Release, October 6, 2021. Available at: https://www.who.int/news/item/06-10-2021-who-recommends-groundbreaking-malaria-vaccine-for-children-at-risk
4 Chandramohan, D. et al. (2021) “Seasonal malaria vaccination with or without seasonal malaria chemoprevention,” New England Journal of Medicine, 385(11), pp. 1005–1017. Available at: https://doi.org/10.1056/nejmoa2026330.
5 Datoo, M.S. et al. (2022) “Efficacy and immunogenicity of R21/matrix-M vaccine against clinical malaria after 2 years’ follow-up in children in Burkina Faso: A phase 1/2B randomised controlled trial,” The Lancet Infectious Diseases [Preprint]. Available at: https://doi.org/10.1016/s1473-3099(22)00442-x.
6 Anon, 2022. New malaria vaccine comes a step closer as experts say it’s ‘the best yet’. The Guardian. Available at: https://www.theguardian.com/global-development/2022/sep/07/malaria-vaccine-truss-cut-funding [Accessed September 26, 2022].
7 O’Neill, P.M.; Barton, V.E.; Ward, S.A. The Molecular Mechanism of Action of Artemisinin—The Debate Continues. Molecules 2010, 15, 1705-1721. https://doi.org/10.3390/molecules15031705
8 Gomes, M., Ribeiro, I., Warsame, M. et al. Rectal artemisinins for malaria: a review of efficacy and safety from individual patient data in clinical studies. BMC Infect Dis 8, 39 (2008). https://doi.org/10.1186/1471-2334-8-39
9 Moore CM, Wang J, Lin Q, Ferreira P, Avery MA, Elokely K, Staines HM, Krishna S. Selective Inhibition of Plasmodium falciparum ATPase 6 by Artemisinins and Identification of New Classes of Inhibitors after Expression in Yeast. Antimicrob Agents Chemother. 2022 May 17;66(5):e0207921. doi: 10.1128/aac.02079-21. Epub 2022 Apr 25. PMID: 35465707; PMCID: PMC9112895.
10 Wang J, Xu C, Wong YK, Ma N, Liao FL, Jiang T, Tu Y. 2020. Triple artemisinin-based combination therapies for malaria: proceed with caution. Lancet 396:1976. 10.1016/S0140-6736(20)32400-4. [PubMed] [CrossRef] [Google Scholar]
11 Tilley L, Straimer J, Gnädig NF, Ralph SA, Fidock DA Artemisinin action and resistance in Plasmodium falciparum. Trends Parasitol. 2016; 32: 682-696
12 WHO World malaria report 2019. https://www.who.int/publications/i/item/9789241565721 Date: Dec 4, 2019 Date accessed: 29th October, 2022
13 Hanboonkunupakarn B, Tarning J, Pukrittayakamee S, Chotivanich K. Artemisinin resistance and malaria elimination: Where are we now? Front Pharmacol. 2022 Sep 23;13:876282. doi: 10.3389/fphar.2022.876282. PMID: 36210819; PMCID: PMC9538393.
Malaria section authored by Herbie Loening